Description
A typical solar panel uses about 2 - 2.5 grams of Si per watt.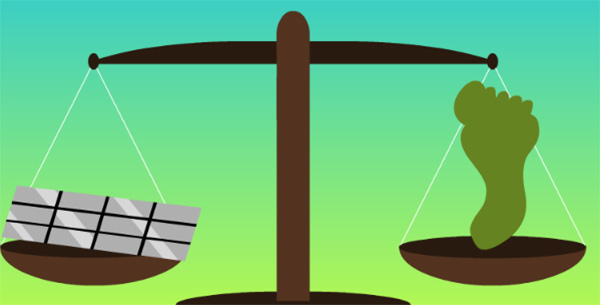
Introduction
Solar panels, often called photovoltaic panels, have grown in popularity due to the increasing demand for renewable energy sources. These panels have been pivotal in transitioning from fossil fuel reliance to greener energy solutions.
Brief Overview of Solar Panels
Solar panels are devices that convert sunlight into electricity. They are commonly made up of many solar cells linked together to produce a specific
power output. These panels have been incorporated in various setups, from residential rooftops to vast solar farms. A typical residential solar panel size is about 1.65 m x 1 m, with varying thickness, usually around 35mm to 40mm. The
efficiency of these panels generally ranges from 15% to 20%, though higher efficiencies are possible with premium panels. The
lifetime of a standard solar panel can vary, but many manufacturers offer warranties for 25 years, indicating their longevity.
Role of Polysilicon in Solar Technology
Polysilicon or poly-Si, is a key material used in the production of solar cells. It is derived from quartzite, a type of quartz sandstone rock. In the context of solar panels, polysilicon serves as the foundational material for the creation of the cells that absorb sunlight. The
quality of polysilicon can directly influence the efficiency and longevity of the solar cells. A solar cell made from high-purity polysilicon can achieve efficiencies of over 20%, but these numbers can vary depending on manufacturing processes and other materials used. As for the
cost, the price of polysilicon has fluctuated over the years due to market dynamics, technological advancements, and supply chain factors. In 2020, for instance, the cost was approximately $8 to $11 per kilogram.
The choice of polysilicon also impacts the panel's
performance over its lifespan. While most panels retain about 80% of their capacity after 25 years, the type and quality of the polysilicon used can alter this figure.
In conclusion, understanding the role and specifications of polysilicon is paramount when assessing the functionality and longevity of solar panels. As the solar industry continues to evolve, so too will the methods and materials used in panel production.
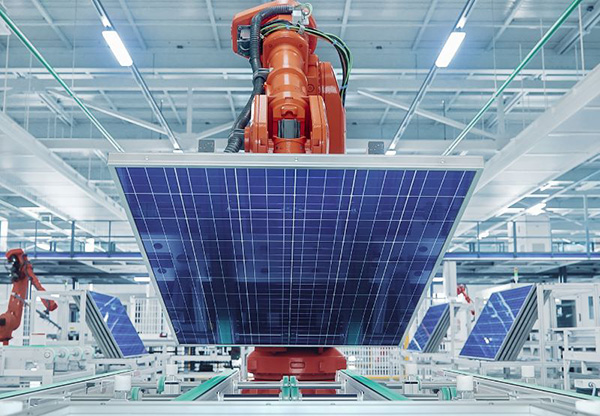
History and Evolution of Polysilicon in Solar Cells
The journey of polysilicon in the realm of solar cells is both fascinating and illuminating. Charting the course of its evolution provides invaluable insights into the technological advancements and economic considerations that have shaped the solar industry.
Early Usage and Advancements
In the mid-20th century, the semiconductor industry was burgeoning, and with it, the initial demand for silicon material, primarily
polysilicon, was established. The 1950s saw the birth of the first silicon solar cells, boasting an
efficiency of about 6%. This was a remarkable leap from selenium solar cells, which only had an efficiency of 0.5%.
One of the critical early milestones was the use of the Czochralski process. This method allowed for the production of single-crystal silicon, leading to improved solar cell efficiency. By the late 1970s, driven by the energy crisis and increased research funding, solar cell efficiencies had climbed to about 10%. Additionally, the
cost per watt of solar-generated power began its steady descent, dropping from a hefty $76.67 in 1977 to around $10 by the early 1980s.
Transition from Monocrystalline to Multicrystalline Silicon
Monocrystalline silicon, derived from a single crystal, was the standard in early solar cell production. However, the production process was not only time-consuming but also expensive. As the demand for solar cells grew, a need for a more cost-effective material emerged.
This demand led to the rise of
multicrystalline silicon or multi-Si. While monocrystalline panels are known for their black hue and higher efficiency (typically between 20% to 22%), multicrystalline panels, recognizable by their bluish hue, are less efficient, ranging from 15% to 17%. However, their production process is simpler and more economical.
By the 1990s, multicrystalline silicon became a dominant player in the market due to its balance between
cost and efficiency. It's worth noting that as of 2019, the average global price of polysilicon was around $9 per kilogram, a drastic reduction from prices seen in the 2000s, which hovered around $450 per kilogram.
In sum, the evolution of polysilicon in solar cells reflects a delicate dance between technological advancement and market economics. The pursuit of higher efficiencies and lower costs continues to drive innovation in the industry.
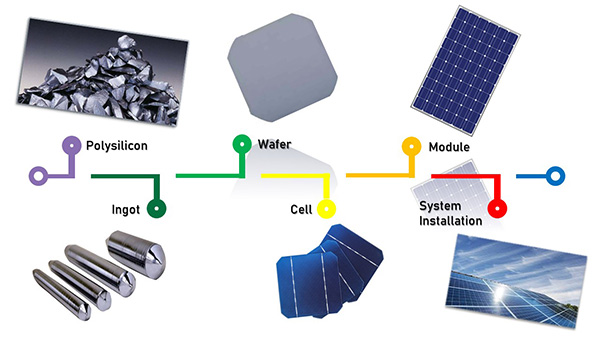
Extraction and Production of Polysilicon
The journey of converting sand into a highly purified polysilicon, suitable for solar cells, is a testament to human ingenuity and technological prowess. Diving into the extraction and production processes unravels the complexity behind the seemingly simple solar panels we see today.
Methods of Extracting Silicon from Raw Materials
At its core, silicon is derived from sand, or more precisely, quartz, which is silicon dioxide (SiO2). The extraction process involves a series of steps to transform this abundant material into a usable form for solar cells.
- Quartz Mining: This is the initial step, where high-purity quartz is mined from the earth. This quartz is then transported to processing plants for further purification.
- Reduction to Metallurgical-Grade Silicon (MG-Si): In this phase, the quartz is reduced in electric arc furnaces. By combining quartz with carbon materials, like coke and coal, silicon is produced, resulting in a product that is about 98% pure. While this purity level is sufficient for certain industries, solar cell production requires an even higher purity.
- Purification: This is the most crucial step in terms of quality. To achieve the desired purity of 99.9999%, MG-Si is refined further using methods like the Siemens process. The goal here is to eliminate impurities such as boron and phosphorus, which can severely hamper the efficiency of solar cells.
Polysilicon Production Process
Once we have a sufficiently pure form of silicon, the next challenge is to transform it into a usable form for solar cell production - polysilicon.
- Siemens Process: This is the most widely used method for producing polysilicon. In this process, a rod of high-purity silicon is exposed to gaseous silicon compounds. As these compounds decompose, they deposit additional silicon onto the rod, increasing its size. The cost of this process can vary, but in 2019, it hovered around $14 per kilogram of produced polysilicon.
- Fluidized Bed Reactor (FBR) Process: An alternative to the Siemens process, the FBR method is gaining traction due to its lower costs and comparable quality of produced polysilicon. Here, silicon seed particles are suspended in a reactor while silicon gas compounds are introduced. As these compounds break down, they deposit onto the seed particles, forming granular polysilicon. This method can cut production costs by up to 30% compared to the Siemens process.
In conclusion, the extraction and production of polysilicon are intricate processes, rooted in advanced science and engineering. As the solar industry continues to expand, refining these processes to achieve better efficiencies at reduced costs remains a paramount objective.
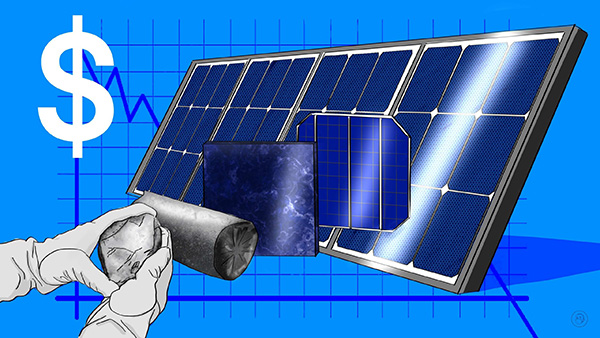
Quantitative Analysis
Delving into the quantitative analysis of polysilicon in solar panels reveals fascinating insights. This quantitative lens helps us grasp not only the material efficiency but also the potential avenues for optimization in the industry.
Average Amount of Polysilicon per Solar Panel
A standard solar panel, often referred to as a 60-cell module, measures about 1.6 meters by 1 meter. On average, the amount of polysilicon used in such a panel is roughly 11-12 grams per watt. Given that a typical panel has a
power output of 300 watts, this translates to approximately 3.3 to 3.6 kilograms of polysilicon per panel. However, these figures can fluctuate based on the design and efficiency of the panel.
Factors Influencing Polysilicon Usage
Several factors dictate the quantity of polysilicon employed in a solar panel:
- Panel Efficiency: Higher efficiency panels tend to use polysilicon more optimally. Thus, as efficiency increases, the polysilicon requirement per watt often decreases.
- Manufacturing Process: The method used to produce the silicon wafers can influence the amount of material wasted. Cutting-edge techniques aim to reduce wastage and optimize material use.
- Thickness of Solar Cells: The advent of new technologies has led to thinner solar cells. A decade ago, the standard thickness was around 200 micrometers, but with advancements, we now have cells as thin as 160 micrometers or even less. Thinner cells mean less polysilicon is used for the same area.
- Market Demand and Price: As with any commodity, the price of polysilicon influences its usage. When prices are high, manufacturers might lean towards optimizing usage and reducing waste.
Trends Over the Years
Historical data unveils an intriguing trajectory in polysilicon use:
- Decrease in Polysilicon per Watt: Over the past two decades, the amount of polysilicon used per watt has seen a steady decline, dropping by approximately 50% since the early 2000s.
- Rising Solar Panel Efficiency: The average efficiency of solar panels has been on an upward trajectory. In the early 2000s, average efficiencies were around 12-15%. Fast forward to the present, and many commercial panels boast efficiencies exceeding 20%.
- Reduction in Costs: The cost of polysilicon has witnessed a dramatic reduction. From prices above $400 per kilogram in the mid-2000s, the market saw a drastic fall to around $10 per kilogram by the end of 2019.
In essence, the journey of polysilicon in solar panels, examined quantitatively, reflects an industry continuously refining its practices. This persistent optimization, driven by both technological advancements and market forces, has been instrumental in making solar energy more accessible and affordable.
Impact of Polysilicon Thickness on Efficiency
Understanding the relationship between the thickness of polysilicon and the efficiency of solar cells sheds light on a fundamental aspect of solar technology. This relationship is vital not only from a performance perspective but also for its implications on material usage and production costs.
How Thickness Affects Solar Cell Performance
The thickness of polysilicon in a solar cell holds significant sway over its performance:
- Light Absorption: Polysilicon's primary role in a solar cell is to absorb sunlight and convert it into electricity. A thicker layer might absorb more light, especially in the infrared spectrum. However, if the cell is too thick, some of the absorbed light might recombine before it's converted into electricity, leading to losses.
- Charge Collection: Once sunlight is absorbed, it generates electron-hole pairs that need to be separated and collected efficiently. In excessively thick cells, these charges may recombine before reaching the electrodes, reducing the cell's efficiency.
- Material Defects: Thicker layers are more prone to defects, which can serve as recombination centers. These defects hinder the flow of charges and can significantly impact the cell's performance.
- Mechanical Properties: Thicker cells are generally more robust and less susceptible to breakage during handling and installation. However, they also mean increased material costs.
Optimal Thickness for Maximum Efficiency
Determining the ideal thickness is a balance between maximizing light absorption and minimizing charge recombination:
- Trends in Thickness: A couple of decades ago, standard polysilicon solar cells had a thickness of about 200-300 micrometers. Modern advancements have seen this number drop, with many manufacturers now producing cells as thin as 150 micrometers or even less.
- Performance Peak: Research indicates that there's a performance peak in the range of 100-200 micrometers for many cell designs. Within this range, cells absorb light efficiently while also minimizing charge recombination.
- Future Directions: With the advent of new materials and manufacturing techniques, like PERC (Passivated Emitter Rear Cell), the optimal thickness might further decrease. Simultaneously, techniques like light trapping could allow even thinner cells to absorb as much light as their thicker counterparts.
In conclusion, the thickness of polysilicon in solar cells is a critical parameter. As technology progresses and the industry continues its pursuit of higher efficiencies at reduced costs, the delicate balance between thickness and performance remains a central theme in solar research and development.
Environmental and Economic Implications
Exploring the environmental and economic implications of polysilicon provides a comprehensive understanding of its broader impact beyond its technical utility in solar panels. These insights encompass the very essence of why solar technology remains at the forefront of sustainable energy solutions, while also highlighting the complexities of its production and market dynamics.
Polysilicon Production and Carbon Footprint
The process of manufacturing polysilicon, while integral to renewable energy production, does come with its own environmental considerations:
- Energy Intensive Process: Producing polysilicon, especially through the traditional Siemens process, is energy-intensive. This process requires temperatures of over 1,000°C. Depending on the source of this energy, the carbon footprint can be significant. For instance, if coal powers the production plants, the emissions are considerably higher.
- Waste Generation: The production of polysilicon leads to waste materials, including silicon tetrachloride. Managing and recycling this waste is crucial to prevent environmental damage.
- Carbon Reduction Over Lifecycle: Despite the carbon emissions during production, the use of polysilicon in solar panels results in a net reduction of carbon over the panel's lifecycle. A solar panel typically offsets its carbon footprint within a few years of operation and goes on to produce carbon-free electricity for the remainder of its lifecycle.
- Transition to Greener Production: Recognizing the environmental implications, many manufacturers are transitioning to more sustainable energy sources for polysilicon production, further reducing its carbon footprint.
Cost Implications and Market Dynamics
The economic landscape surrounding polysilicon is ever-evolving and deeply intertwined with its environmental implications:
- Production Costs: Historically, the cost of polysilicon production was a significant portion of the overall cost of a solar panel. However, with technological advancements and economies of scale, these costs have been steadily decreasing.
- Market Volatility: The polysilicon market has experienced volatility due to supply-demand dynamics. Factors like trade policies, production capacity expansions, and technological advancements have led to price fluctuations.
- Economic Drivers: The push towards greener production methods, while environmentally commendable, does come with increased costs. However, many regions are offsetting these with incentives, subsidies, or carbon credits, recognizing the long-term benefits of sustainable polysilicon production.
- End-of-Life Costs: Solar panels have a lifespan of 20-30 years. As panels reach the end of their life, recycling or disposing of them becomes an economic consideration. Initiatives are emerging to recycle and reclaim valuable materials like polysilicon, mitigating both environmental and economic implications.
In summary, while polysilicon serves as a cornerstone of solar technology, its environmental and economic ramifications are multifaceted. Balancing the immediate environmental costs of its production with the long-term benefits of renewable energy generation remains a central challenge for the industry. Equally, navigating the intricate economic terrain, shaped by market, technological, and policy forces, is pivotal for the continued growth and sustainability of solar energy.