Description
Higher temperatures reduce solar cell efficiency and energy output, while lower temperatures tend to improve them.

Basics of Solar Cell Operation
Solar cells, also known as photovoltaic (PV) cells, convert sunlight directly into electricity. This process relies on the photovoltaic effect, a physical and chemical phenomenon, which occurs when a material generates an electric voltage or current upon exposure to light.
Fundamental Principles of Solar Cells
The core principle of solar cell operation centers around the photovoltaic effect. Semiconducting materials, typically silicon, form the basis of most solar cells. When sunlight hits the solar cell, the solar energy excites electrons in the semiconductor, freeing them from atoms and allowing them to flow through the material, creating an electrical current. The efficiency of this process significantly influences the power output, which varies based on the solar cell type and its material properties.
Types of Solar Cells and Their Characteristics
Solar cells come in various types, each with distinct characteristics, advantages, and disadvantages. Here, we will discuss three primary types:
Monocrystalline Solar Cells
- Materials and Quality: Made from single-crystal silicon, monocrystalline panels are typically more efficient and more expensive.
- Performance and Efficiency: They offer high efficiencies (about 15-20%) and perform better in low light conditions compared to other types.
- Dimensions and Aesthetics: These cells have a uniform dark look and rounded edges.
- Lifespan and Durability: Monocrystalline panels usually have a longer lifespan, often exceeding 25 years.
- Cost Implications: While the initial investment is higher, their longevity and efficiency can provide better value over time.
Polycrystalline Solar Cells
- Materials and Quality: Constructed from multiple silicon crystals, these are less efficient but also less expensive.
- Performance and Efficiency: Polycrystalline cells typically have efficiencies around 13-16%.
- Dimensions and Aesthetics: They have a speckled blue color and do not require as much silicon as monocrystalline cells.
- Lifespan and Durability: They generally have a slightly shorter lifespan than monocrystalline cells.
- Cost Implications: Lower upfront costs make them a budget-friendly option for residential use.
Thin-Film Solar Cells
- Materials and Quality: Made by depositing one or more thin layers of photovoltaic material onto a substrate. These can be made from a variety of materials, including cadmium telluride (CdTe) or amorphous silicon.
- Performance and Efficiency: Thin-film cells generally have lower efficiencies (about 10-13%) compared to crystalline silicon cells.
- Dimensions and Flexibility: These cells are lightweight and can be made flexible, offering new application possibilities.
- Lifespan and Durability: They tend to degrade faster than crystalline silicon cells, reducing their lifespan.
- Cost and Installation: The production process for thin-film cells is simpler and potentially cheaper, but the cost per watt might be higher due to lower efficiencies.
For a comprehensive understanding of the different types and their specifications, refer to the
Wikipedia page on Solar Cells. The choice between these types often boils down to balancing efficiency, cost, and space requirements. Each type has its unique set of parameters that can influence the decision based on the specific application, location, and budget constraints.
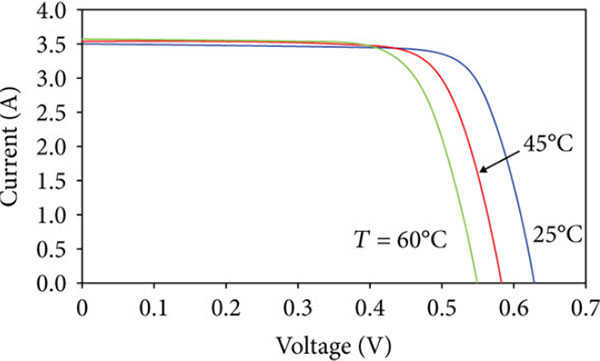
The Science Behind Temperature and Solar Cell Efficiency
Understanding the impact of temperature on solar cell efficiency is crucial for maximizing the performance of photovoltaic systems. Solar cell efficiency, the percentage of solar energy converted into electrical energy, varies with temperature changes. Typically, as temperature increases, solar cell efficiency decreases.
How Temperature Influences Photovoltaic Materials
Photovoltaic materials like silicon exhibit changes in their electrical properties when exposed to different temperatures. At higher temperatures, silicon's bandgap - the energy difference between the valence band and conduction band - narrows. This narrowing bandgap reduces the energy required for electrons to jump to the conduction band, increasing the rate of electron-hole pair generation. However, this also lowers the voltage output and thus the overall efficiency.
For instance, standard silicon solar cells might start with an efficiency of around 18-22% at 25°C (room temperature). But the efficiency can drop by about 0.4% for every degree increase in temperature. This change in efficiency is crucial in hot climates where solar panels can reach temperatures well above 45°C, leading to significant drops in performance.
Temperature Coefficients and Their Role in Solar Cell Performance
Each solar cell material comes with a specified temperature coefficient, a parameter that quantifies the change in power output or efficiency due to temperature variation. For example, a solar cell with a temperature coefficient of -0.5% per °C would produce 0.5% less power for each degree Celsius rise in temperature.
Power Output and Temperature Coefficient
- Understanding the Coefficient: The temperature coefficient typically ranges from -0.2% to -0.5% per °C for most crystalline silicon solar cells.
- Impact on Power Output: A solar panel rated at 300 watts with a temperature coefficient of -0.4%/°C would see a reduction of 1.2 watts in output for every degree increase in temperature above 25°C. This change can significantly impact performance over time, especially in warmer climates.
Cost and Efficiency Considerations
- Balancing Cost and Temperature Tolerance: Some solar cells, like monocrystalline, might have better temperature tolerance but at a higher cost. This factor becomes a key consideration when evaluating the long-term efficiency and cost-effectiveness of a solar installation.
- Selecting the Right Panel: Users must choose panels with lower temperature coefficients in regions with high temperature ranges to ensure consistent power output.
For deeper insights into solar cell materials and their temperature coefficients, the
Wikipedia page on Photovoltaic System offers valuable information.
The interaction between temperature and solar cell efficiency is a pivotal aspect of solar panel design and deployment. Understanding these dynamics helps optimize solar power systems for various climates and conditions, ensuring reliability, efficiency, and cost-effectiveness.
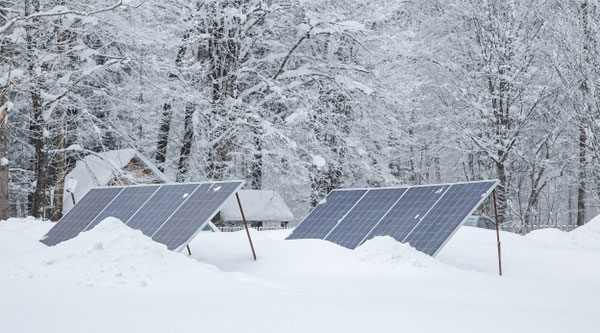
Impact of High Temperatures on Solar Cells
High temperatures can significantly affect the performance and longevity of solar cells. The intricate relationship between solar cell materials, temperature, and efficiency is a key focus in the field of photovoltaic research and development.
Effects of Heat on Solar Panel Efficiency
High temperatures primarily reduce the efficiency of solar panels. As the temperature rises, the semiconductor material within the solar cells generates more energy from the same amount of sunlight, which ironically reduces the voltage output and overall efficiency.
- Decrease in Efficiency: A solar panel's efficiency typically decreases by about 0.4% to 0.5% with each 1°C rise in temperature above 25°C (the standard testing condition for solar panels). For instance, if a panel operates at an efficiency of 20% at 25°C, its efficiency might drop to around 18% at 40°C. This reduction can significantly impact the energy yield, especially in hot climates.
- Power Output Loss: Considering a solar panel with a power rating of 300 watts, a temperature increase from 25°C to 35°C could reduce its output by about 15 watts (5%), affecting the overall power generation, especially during peak sun hours.
Material Degradation Due to High Temperatures
High temperatures not only affect immediate performance but also accelerate the aging process of solar panel components, leading to shorter lifespans and degraded performance over time.
- Thermal Cycling: Repeated heating during the day and cooling at night can cause thermal cycling, leading to physical stress and eventual microcracks in the solar cells.
- UV Exposure and Heat: Continuous exposure to UV light and high temperatures can degrade the encapsulant and backsheet materials, reducing the panel's ability to protect against moisture and mechanical damage.
Cooling Strategies for Solar Panels
To mitigate the impact of high temperatures, various cooling strategies are essential. Effective cooling maintains efficiency and extends the lifespan of solar panels.
- Passive Cooling: This involves designing panels and installations to dissipate heat naturally. Techniques include using heat sinks, spacing panels for air circulation, and choosing materials with higher heat tolerance.
- Active Cooling: More sophisticated approaches use water or air to cool the panels actively. These systems can significantly reduce temperatures but add complexity and cost to the solar installation.
Considering the temperature impact is crucial when planning and installing photovoltaic systems. For more information on how temperature affects solar cells and related technologies, the
Wikipedia page on Solar Cell Efficiency provides detailed insights.
Understanding these impacts guides the selection of appropriate solar panel types, installation methods, and cooling strategies, ensuring that the solar power system remains efficient, reliable, and cost-effective throughout its operational life.
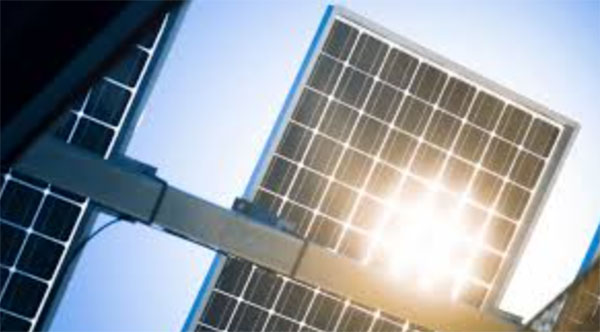
Performance in Cold Conditions
Contrary to common belief, solar cells can perform exceptionally well in cold conditions. Cold climates can offer some advantages for solar cell operation, albeit with a few challenges.
Efficiency Boost at Lower Temperatures
Solar cells, mostly silicon-based, tend to operate more efficiently at lower temperatures. This efficiency increase is due to the nature of semiconductor materials from which solar cells are made.
- Improved Voltage and Efficiency: As temperatures drop, the voltage output of solar cells increases, which can lead to higher overall efficiency. A solar panel that operates at 15-20% efficiency in moderate temperatures might see a slight increase in this efficiency in colder weather.
- Power Output Considerations: Even though the daylight hours are shorter in winter, the power output during these hours can be higher due to improved efficiency. For example, a 300-watt panel might produce more power per hour of sunlight in cold conditions than in hot conditions, despite the shorter days.
Challenges in Cold Climates
While cold temperatures can improve efficiency, other factors associated with cold climates can pose challenges to the effective operation of solar panels.
- Snow Accumulation: Snow cover on panels can block sunlight, significantly reducing power generation. The design and installation of panels in cold regions often need to account for snow, including tilt angle and ease of clearing snow.
- Reduced Daylight Hours: Shorter daylight hours during winter months result in fewer hours of sunlight, limiting the total daily power production.
- Material Contraction: Extremely low temperatures can cause materials to contract, potentially affecting the structural integrity of the panels and their mounting systems. Using materials that can withstand these changes without damage is important for maintaining long-term performance.
Benefits and Challenges of Operating Solar Cells in Cold Climates
Cold climates offer a unique setting for solar panels, providing both benefits and challenges:
- Benefits: Enhanced efficiency and lower degradation rates are key benefits. Solar panels in colder climates might have a longer lifespan compared to those in hotter climates, where high temperatures can accelerate aging and efficiency loss.
- Challenges: Besides snow and shorter daylight hours, maintenance in cold weather can be more challenging, and installations may require more robust mounting systems to handle snow loads and wind.
For more detailed information on solar cells' performance in different climates, the
Wikipedia page on Photovoltaic System can be a valuable resource.
Solar panels in cold climates can offer efficient and effective performance, with some considerations for the unique challenges posed by these environments. By addressing these challenges, solar energy can be a reliable power source even in the coldest regions.
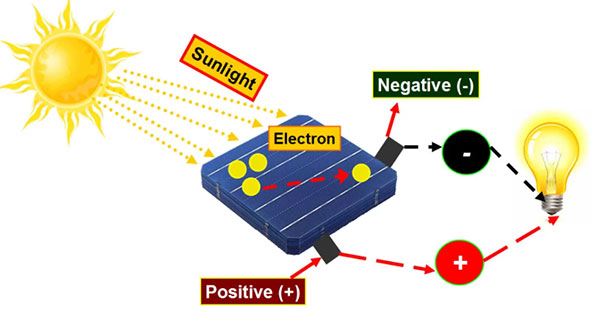
Temperature Regulation and Solar Cell Longevity
Maintaining optimal temperatures is crucial for maximizing the longevity and efficiency of solar cells. Excessive heat can lead to physical degradation of solar panels, reducing their operational life and efficiency. Managing temperature effectively can significantly enhance both the performance and lifespan of these devices.
Role of Temperature in Solar Cell Degradation
Solar cell degradation is a critical factor in determining the lifespan and performance of solar panels.
- Impact on Lifespan: Elevated temperatures can accelerate the aging process of various components of solar panels. For instance, for every 10°C increase in temperature, the rate of aging doubles, potentially halving the lifespan of the solar panel.
- Efficiency Loss: Prolonged exposure to high temperatures can lead to a decrease in power output. Research shows that solar panel efficiency can decrease by 0.25% to 0.5% for every 1°C increase in temperature above 25°C.
Techniques for Maintaining Optimal Operating Temperatures
Various methods can help in maintaining the solar panels at optimal temperatures, thereby enhancing their performance and longevity.
- Cooling Systems: Active cooling systems, like fluid-based cooling, can effectively remove excess heat from solar panels. These systems can be integrated into the solar panel design but may add to the overall cost and complexity.
- Improved Panel Design: Using materials that reflect more sunlight or dissipate heat more effectively can help in keeping the solar cells cool. Panels designed with a larger gap between them and the mounting surface can also promote better air circulation, aiding in cooling.
- Coatings and Materials: Special coatings that enhance heat dissipation and reduce the solar panel's temperature can be applied. Innovations in materials, such as the development of new semiconductor materials that are more heat resistant, also contribute significantly to temperature regulation.
Innovations in Thermal Management for Solar Cells
Technological advancements are continually emerging in the area of thermal management for solar cells.
- Thermally Conductive Materials: The integration of thermally conductive materials, such as graphene, can enhance heat dissipation from the solar cells, improving their temperature regulation.
- Phase Change Materials (PCMs): PCMs absorb and release thermal energy during the phase change process, helping to regulate the temperature of solar cells effectively. This technology can store excess heat during the hottest part of the day and release it when the temperature drops.
- Advanced Cooling Technologies: Researchers and companies are exploring high-efficiency cooling technologies, such as microfluidic cooling layers, which can be integrated directly into the solar panel structure, providing efficient heat dissipation without significant energy consumption or added bulk.
For a deeper understanding of the relationship between temperature and solar cell efficiency, the
Wikipedia page on Solar Cell Efficiency offers comprehensive information.
Proper temperature regulation not only enhances the immediate power output of solar cells but also plays a critical role in determining their long-term reliability and operational life. By incorporating innovative cooling techniques and materials, the solar industry continues to improve the viability and sustainability of solar energy solutions.