Description
The difference between a photovoltaic cell and a solar cell primarily lies in their scope and application. A photovoltaic cell is a type of solar cell specifically designed to convert sunlight into electrical energy through the photovoltaic effect. Essentially, all photovoltaic cells are solar cells, but not all solar cells are strictly used for generating electricity. Some solar cells might find use in other light-related applications like light sensors.

Basic Concepts
Understanding the fundamental concepts of solar and photovoltaic cells is essential in grasping their functionality, applications, and advancements in renewable energy technology.
Definition of Solar Cells
Solar cells, often referred to as "photovoltaic cells," are devices that convert sunlight directly into electricity. These cells are made primarily from materials that exhibit the photovoltaic effect, such as silicon. When sunlight strikes a solar cell, it agitates electrons in the cell's material, creating a flow of electrical current. Solar cells are the building blocks of larger solar panels, which people use in various applications ranging from residential solar power systems to large solar farms.
Definition of Photovoltaic Cells
A photovoltaic cell is a specialized semiconductor diode that converts visible light into direct current (DC). While all solar cells are photovoltaic devices, not all photovoltaic cells are necessarily solar cells. Photovoltaic cells can respond to other forms of light beyond the solar spectrum, making them useful in a broader range of applications, such as calculators and spacecraft.
Historical Development of Solar and Photovoltaic Technologies
Solar and photovoltaic technology has made significant strides since its inception in the 19th century. French physicist Edmond Becquerel first discovered the photovoltaic effect in 1839, laying the groundwork for future developments. Bell Labs in the U.S. then pioneered the first practical silicon solar cell in the 1950s, achieving about 6% efficiency and kickstarting modern solar energy technology.
Since then, solar cell efficiency has soared, with commercial cells now typically delivering between 15% and 20% efficiency and some lab samples reaching beyond 26%. This progress is due to improvements in materials, design, and production techniques.
Alongside efficiency improvements, solar technology has become more affordable. Initially expensive and used mainly in space exploration, economies of scale and technological advancements have substantially lowered costs, positioning solar power as a viable competitor to fossil fuels globally.
Today, emerging technologies like perovskite and organic photovoltaic cells are under investigation, promising potentially even higher efficiencies and lower production costs, heralding a new era of versatile and sustainable solar energy applications.
Solar and photovoltaic technologies continue to evolve, promising a future where renewable energy sources might dominate global energy production, reducing dependence on fossil fuels and mitigating the impact of climate change. Their ongoing development, marked by milestones in efficiency, cost, and applicability, remains crucial to achieving a sustainable energy future.
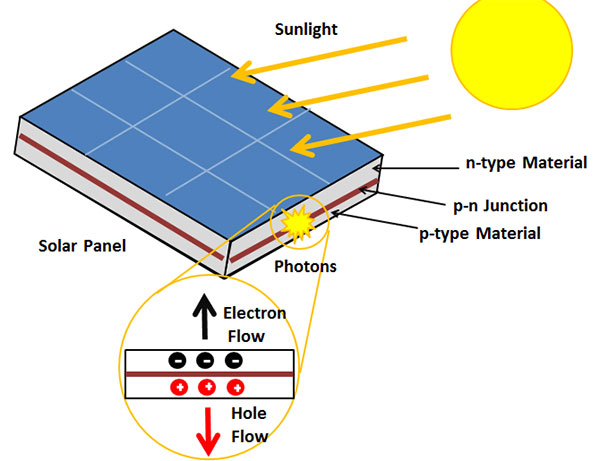
Design and Composition
The design and composition of solar and photovoltaic cells are critical in their operation, efficiency, and applications. By understanding their material make-up and structure, we can appreciate how these cells harness the sun's energy and the technological advancements that make them more efficient and cost-effective.
Material Composition of Solar Cells
Solar cells typically use silicon, a semiconductor, as their primary material. Silicon's atomic structure makes it ideal for absorbing sunlight and converting it into electricity. There are primarily two types of silicon used in solar cells:
- Monocrystalline Silicon: Known for its purity and high efficiency, monocrystalline silicon is made from single-crystal silicon. Solar cells made from this material often achieve efficiencies above 20%, but they come with higher production costs. Their distinctive uniform dark look and rounded edges make them easily recognizable.
- Polycrystalline Silicon: Unlike monocrystalline, polycrystalline silicon comes from melting multiple silicon crystals together. These solar cells are simpler and cheaper to produce but typically offer slightly lower efficiencies, usually around 15-17%. They can be identified by their speckled blue appearance and square shape.
Other materials like Cadmium Telluride (CdTe) and Copper Indium Gallium Selenide (CIGS) also feature in thin-film solar cells, offering different efficiencies, costs, and applications.
Material Composition of Photovoltaic Cells
Photovoltaic cells, while also primarily using silicon, can include a variety of other materials:
- Thin-Film Photovoltaic Cells: These use materials like amorphous silicon, CIGS, and CdTe. They are cheaper to produce than crystalline silicon cells but generally offer lower efficiencies, typically around 10-12%. Their thin and flexible nature allows for integration into a variety of surfaces, such as building windows and flexible solar panels.
- Emerging Materials: New materials such as perovskites are being researched for their potential to offer high efficiencies at lower manufacturing costs. Their ability to be printed using simple techniques holds the promise for cost-effective production and innovative applications.
Structural Differences and Similarities
The structural design of solar and photovoltaic cells largely depends on the materials and intended application:
- Layered Composition: Both solar and photovoltaic cells usually consist of multiple layers. These layers include the semiconductor material, anti-reflective coating to absorb more light, and a backing material for protection and insulation.
- Electrical Configuration: Cells are interconnected to form a module, which can then be assembled into larger arrays. The electrical configuration is crucial in determining the overall power output and voltage of the solar panel or photovoltaic system.
- Size and Shape Variations: The size and shape of solar cells can vary based on application needs. Residential and commercial solar panels typically require larger, more efficient cells, whereas portable devices might use smaller, flexible thin-film cells.
In conclusion, the design and composition of solar and photovoltaic cells are essential in determining their efficiency, cost, and suitability for different applications. Advances in materials science and manufacturing techniques continue to drive improvements in these aspects, making solar energy a more accessible and viable source of renewable energy.
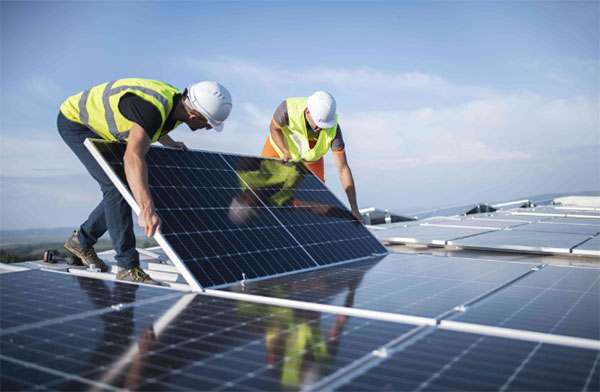
Working Principles
Understanding the working principles of solar and photovoltaic cells is key to comprehending how they convert sunlight into electrical energy. Despite their similar objectives, the mechanisms they use can differ, which influences their efficiency, cost, and application.
How Solar Cells Work
Solar cells convert sunlight into electricity using the photovoltaic effect. The process involves several key steps:
- Absorption of Sunlight: The solar cell's semiconductor material, typically silicon, absorbs light. Each photon of light energy that the silicon absorbs frees an electron, creating a hole.
- Creation of Electron-Hole Pairs: Each free electron leaves behind a hole in the atomic structure of the semiconductor. This separation of charge carriers creates an electrical imbalance within the cell.
- Generation of Electrical Current: An external circuit connects the top and bottom of the solar cell. When light agitates the electrons, and they flow towards the positive side of the cell, this movement generates an electrical current.
- Efficiency Factors: The efficiency of a solar cell depends on the materials used, the quality of the semiconductor, and the amount of sunlight absorbed. Typical commercial solar cells have efficiencies between 15% and 20%, but some laboratory cells have reached over 26%.
How Photovoltaic Cells Convert Light into Electricity
Photovoltaic cells, while functioning on the same basic photovoltaic principle as solar cells, can have different compositions and designs that affect their light conversion process:
- Broad Spectrum Light Absorption: Advanced photovoltaic cells are designed to absorb a broader range of the light spectrum, including ultraviolet and infrared, which can potentially increase electricity output.
- Layered Cell Structures: Some photovoltaic cells use multiple layers of different semiconductor materials to capture different wavelengths of light, which can enhance overall efficiency.
- Efficiency and Cost: While traditional photovoltaic cells made of crystalline silicon typically show higher efficiency, newer materials like thin-film solar cells and perovskite cells offer the promise of lower costs and greater flexibility, albeit often at lower efficiency rates.
Comparative Analysis of Working Mechanisms
When comparing solar cells and photovoltaic cells:
- Material Efficiency: Monocrystalline and polycrystalline silicon cells, common in both solar and photovoltaic cells, offer high efficiencies but at a higher cost. Thin-film and perovskite technologies, while more affordable and versatile, usually provide lower efficiencies.
- Application Suitability: The choice between solar and photovoltaic cells often depends on the application. High-efficiency monocrystalline cells are preferable for areas with limited space, like rooftops, whereas thin-film cells can be ideal for large-scale installations or integrated solar products due to their flexibility and lower costs.
- Technological Advancements: Continuous research is improving the efficiencies of both types of cells, reducing costs, and expanding their potential applications. Emerging technologies like bifacial solar panels, which capture sunlight from both sides, and floating solar farms, highlight the innovative approaches enhancing the effectiveness of solar energy capture and use.
In summary, both solar and photovoltaic cells serve the crucial role of converting solar energy into electrical power, but they do so with varying materials, structures, and efficiencies. Technological advancements and research continue to refine these methods, aiming to make solar power a more accessible and efficient energy source globally.
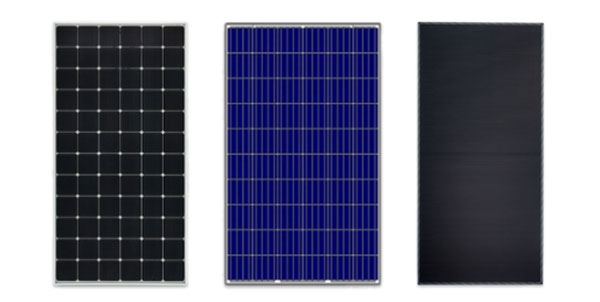
Types and Variations
The solar and photovoltaic (PV) cell industry has evolved significantly, offering various types and technologies. Each type comes with its unique set of characteristics in terms of efficiency, cost, and applications. Understanding these differences helps in selecting the right technology for specific needs.
Different Types of Solar Cells
Solar cells have diversified into several types, each with distinct features and applications:
- Monocrystalline Silicon Cells: Known for their high efficiency (about 20% and above) and long lifespan (usually over 25 years), these cells are ideal for applications where space is limited. However, they come at a higher cost compared to other types.
- Polycrystalline Silicon Cells: These cells offer slightly lower efficiency (around 15-17%) but at a lower cost. They are recognizable by their blue, speckled appearance and are commonly used in residential solar installations.
- Thin-Film Solar Cells: These include various materials like amorphous silicon, CdTe, and CIGS, each offering unique advantages. For example, amorphous silicon cells provide good performance in low-light conditions. Thin-film cells are generally more affordable but have lower efficiencies (around 10-12%) and shorter lifespans.
- Biohybrid Solar Cells: These innovative cells use organic materials and living organisms, like bacteria, to harvest light more efficiently. While still in the experimental stage, they promise a more sustainable and less costly option for solar energy.
Various Types of Photovoltaic Cells
Photovoltaic cells also vary widely, influenced by their material composition and intended use:
- Conventional Crystalline Silicon PV Cells: These cells are similar to monocrystalline and polycrystalline solar cells, offering reliable performance and efficiency.
- Thin-Film PV Cells: Utilizing the same technology as thin-film solar cells, these PV cells are more flexible and lighter, which is advantageous for certain applications.
- Multijunction PV Cells: Designed for high-efficiency applications, these cells use multiple layers of different semiconductor materials to capture a broader range of the solar spectrum. They are more expensive but can achieve efficiencies over 40%.
- Perovskite PV Cells: Emerging as a low-cost alternative, perovskite cells are gaining attention for their rising efficiencies (over 25% in some cases) and easier manufacturing processes.
Innovations and Advanced Technologies in Solar and Photovoltaic Cells
Innovation in the field is continuous, focusing on improving efficiency, reducing costs, and expanding applications:
- Bifacial Solar Cells: These cells can capture sunlight from both sides, increasing overall efficiency. They are particularly effective in large-scale installations.
- Floating Solar Farms: Utilizing floating platforms, these solar systems are installed on bodies of water, saving land space and benefiting from the cooling effect of water.
- Building-Integrated Photovoltaics (BIPV): BIPV technologies integrate photovoltaic materials into building structures, such as windows or facades, making solar power generation a seamless part of urban architecture.
- Quantum Dot Solar Cells: This technology uses nano-sized semiconductor particles to capture sunlight, offering the potential for higher efficiency rates and the ability to tune the absorption spectrum.
- Transparent Solar Cells: These cells can be integrated into windows and screens, providing a transparent yet power-generating surface, ideal for urban and mobile applications.
Each of these types and innovations in solar and photovoltaic cells comes with its set of parameters, like power output, cost, efficiency, size, and lifespan, influencing their suitability for various applications. As technology advances, we can expect to see newer, more efficient, and cost-effective solutions in this dynamic field.
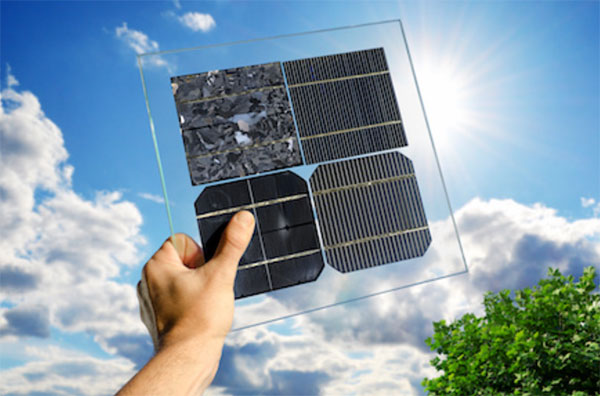
Efficiency and Performance
In the realm of solar and photovoltaic cells, efficiency and performance are critical metrics determining their commercial viability and suitability for various applications. Both these aspects hinge on how effectively these cells convert sunlight into electricity and their operational longevity under different environmental conditions.
Efficiency Metrics in Solar Cells
Solar cell efficiency primarily measures the fraction of sunlight energy that the cell converts into electrical energy. Key metrics include:
- PCE (Power Conversion Efficiency): This is the most common efficiency metric, calculated as the ratio of the electrical power output to the solar power input. Monocrystalline silicon cells typically reach efficiencies around 20%, while polycrystalline silicon cells are slightly lower, generally around 15-17%.
- Fill Factor (FF): This metric indicates the cell's maximum power point (voltage and current) compared to its open-circuit voltage and short-circuit current. Higher fill factors indicate better quality cells.
- Temperature Coefficient: This shows the impact of temperature on the cell's efficiency. A lower temperature coefficient is preferable, as it means the cell's efficiency decreases less under higher temperatures.
Efficiency Metrics in Photovoltaic Cells
Efficiency in photovoltaic cells also revolves around similar metrics but can vary based on the cell technology:
- Quantum Efficiency (QE): QE measures the percentage of photons hitting the cell's surface that contributes to the current. This metric is particularly useful for assessing the performance of new and emerging photovoltaic technologies.
- Energy Payback Time (EPBT): This refers to the time a photovoltaic system needs to generate the same amount of energy that was used to produce it. Advanced thin-film solar cells often exhibit shorter EPBT compared to traditional silicon cells, indicating quicker energy recovery.
Factors Influencing Performance and Efficiency
Several factors can affect the efficiency and overall performance of solar and photovoltaic cells:
- Material Quality: The purity and crystalline quality of silicon or other semiconductor materials significantly influence efficiency. Higher purity typically yields higher efficiency.
- Cell Design: Advanced designs like passivated emitter and rear cell (PERC), bifacial cells, and multi-junction cells can significantly enhance efficiency.
- Incident Light Intensity: Solar cells perform best under strong, direct sunlight. Incidence angle and shading can affect the amount of solar energy reaching the cell.
- Environmental Factors: Temperature, humidity, and soiling (accumulation of dust and dirt) can impact cell performance. For example, higher temperatures can reduce the efficiency of silicon-based cells.
- Ageing and Degradation: Over time, exposure to UV light and weather can degrade materials used in solar cells, reducing their efficiency and lifespan. Most solar cells have a guaranteed life expectancy ranging from 20 to 25 years, with a gradual decrease in efficiency each year.
By understanding and optimizing these efficiency metrics and performance factors, manufacturers and users can ensure maximum output and longevity from their solar and photovoltaic cell installations. Continuous technological advancements are further pushing the boundaries of these metrics, leading to more efficient, cost-effective, and sustainable solar energy solutions.
Applications and Uses
The realm of solar and photovoltaic (PV) technology has witnessed rapid growth, diversifying into a myriad of applications. The flexibility, efficiency, and sustainability of these cells allow for their deployment in various contexts, from small-scale household uses to large industrial installations.
Common Applications of Solar Cells
Solar cells have become increasingly prominent in several key areas:
- Residential and Commercial Buildings: For powering homes and businesses, solar cells provide a cost-effective and environmentally friendly way to reduce electricity bills. Rooftop solar panels are common, with systems designed to meet different power needs.
- Transportation: Solar cells are also gaining traction in the transportation sector, particularly in powering auxiliary systems in cars, buses, and boats. For instance, solar panels can power air conditioning, lights, or entertainment systems in vehicles.
- Remote and Rural Areas: In off-grid locations, where traditional electricity infrastructure is unavailable or costly, solar cells offer a viable solution for basic energy needs, including lighting, heating, and running small appliances.
- Agricultural Applications: Solar technology supports various agricultural operations, such as powering irrigation systems, electric fences, and farm equipment.
- Street Lighting and Public Spaces: Solar-powered street lights and park lighting systems provide an efficient, self-sustaining, and cost-effective lighting solution, particularly in areas with ample sunlight.
Diverse Uses of Photovoltaic Cells
Photovoltaic cells, a subset of solar cells, extend their utility beyond traditional solar power applications:
- Large Scale Power Plants: Solar farms or photovoltaic power stations use vast arrays of PV cells to generate electricity on a large scale, contributing significantly to the grid.
- Portable Power Sources: PV cells are integral in portable solar chargers and power banks, useful for charging mobile devices, particularly during outdoor activities or in emergency situations.
- Space Applications: Satellites and space probes often rely on PV cells for power due to their reliability and ability to function effectively in the harsh conditions of space.
Future Trends in Application and Usage
The future of solar and photovoltaic cell applications appears vibrant and is likely to expand into new domains:
- Building-Integrated Photovoltaics (BIPV): The integration of photovoltaic materials into building materials like glass, roofing, or facades is an emerging trend. BIPV not only generates power but also adds aesthetic value to buildings.
- Wearable Solar Technology: Innovations are leading to the incorporation of PV cells into clothing and accessories, offering a new way to charge devices on the move.
- Artificial Intelligence (AI) and IoT Integration: Combining solar power with AI and the Internet of Things (IoT) paves the way for smarter energy management and optimization in various applications, from smart homes to industrial systems.
- Floating Solar Farms: Installing solar panels on water bodies is an innovative approach to overcoming land constraints, with the added benefit of reduced water evaporation from these bodies.
As solar and photovoltaic technologies evolve, they bring not just improvements in efficiency and affordability but also unlock new, imaginative ways of using energy, completely transforming our approach to energy consumption.
When it comes to choosing from the array of manufacturers in this evolving market,
Tongwei Solar emerges as a leader with its top-tier photovoltaic offerings. Their solar cells and panels, renowned for high efficiency and strong build, are ideal for both home and business settings. Featuring impressive power output and cost-effectiveness, Tongwei’s products are a smart investment for enduring efficiency and performance.